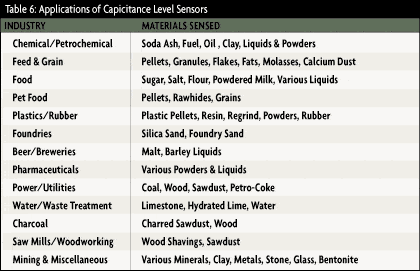
Capacitance level detectors are also referred to as radio frequency (RF) or admittance level sensors. They operate in the low MHz radio frequency range, measuring admittance of an alternating current (ac) circuit that varies with level. Admittance is a measure of the conductivity in an ac circuit, and is the reciprocal of impedance. Admittance and impedance in an ac circuit are similar to conductance and resistance in a direct current (dc) circuit. In this chapter, the term capacitance level sensor will be used
Figure 8-1: Click on figure to enlarge.
instead of RF or admittance.
Table 6 lists some of the industries and applications where capacitance-type level sensors are used.
Theory of Operation
A capacitor consists of two conductors (plates) that are electrically isolated from one another by a nonconductor (dielectric). When the two conductors are at different potentials (voltages), the system is capable of storing an electric charge. The storage capability of a capacitor is measured in farads. As shown in Figure 8-1, the capacitor plates have an area (A) and are separated by a gap (D) filled with a nonconducting material (dielectric) of dielectric constant (K). The dielectric constant of a vacuum is 1.0; the dielectric constants of a variety of materials are listed in Table 7.
The dielectric constant of a substance is proportional to its admittance. The lower the dielectric constant, the lower the admittance of the material (that is, the less conductive it is). Capacitance (C) is calculated as:
C=KA/D
If the area (A) of and the distance (D) between the plates of a capacitor remain constant, capacitance will vary only as a function of the dielectric constant of the substance filling the gap between the plates. If a change in level causes a change in the total dielectric of the capacitance system, because (as illustrated in Figure 8-1B) the lower part of area (A) is exposed to a liquid (dielectric Kl) while the upper part is in contact with a vapor (dielectric Kv, which is close to 1.0), the capacitance measurement will be proportional to level.In the case of a horizontally mounted level switch (Figure 8-2), a conductive probe forms one of the plates of the capacitor (A1), and the vessel wall (assuming it is made from a conductive material) forms the other (A2). An insulator with a low dielectric constant is used to isolate the conductive probe from the housing, which is connected to the vessel wall. The probe is connected to the level sensor via the conductive threads of the housing. Measurement is made

The RF signal results in a minute current flow through the dielectric process material in the tank from the probe to the vessel wall. When the level in the tank drops and the probe is exposed to the even less conductive vapors, the dielectric constant drops. This causes a drop in the capacitance reading and a minute
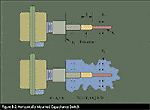
The total area is the combined area of the level sensor probe and the area of the conductive vessel wall (A = A1 + A2), and the distance (D) is the shortest distance between the sensor probe and the vessel wall. Both of these values are fixed. Therefore, when the probe is no longer surrounded by vapors (K1), but by the process material (K2), the resulting capacitance change is directly related to the difference in dielectric constant between the two media:
Change in C=(K2-K1)(A/D)
The sensitivity of a capacitance sensor is expressed in pico-farads (pF). The capacitance unit is the farad, defined as the potential created when a one-volt battery connected to a capacitor causes the storage of one coulomb of electric energy. A pico-farad is one trillionth of that, and the sensitivity of an accurate capacitance detector is 0.5 pF. This is the minimum detectable change in capacitance resulting from a change in dielectric constant (K2 -K1).In most level-sensing applications, the reference material is air (K1 = 1.0). Table 7 gives the K2 values of a variety of process materials. As the dielectric constant of the process material gets close to that of air (K2 for plastic pellets, for example, is 1.1), the measurement becomes more difficult.
Probe Designs
The most common probe design is a stainless steel rod of in. or 1/2 in. diameter, suitable for most non-conductive and non-corrosive materials. The probe is insulated from the housing and bin wall by an low-dielectric insulator, such as Nylon or Ryton. These polymers have maximum operating temperatures of 175-230°C (350-450°F). Ceramics can be used for higher temperature applications or if abrasion resistance is required. For applications where the process material is conductive and corrosive, the probe must be coated with PFA or Kynar.
Some point level sensors are available with build-up immunity, or coating rejection functionality. This is required when the process material is wet or sticky and likely to cause permanent coating. Build-up immunity is provided by the addition of a second active section of probe and a second insulator (Figure 8-3). This second active section (the driven shield) is driven at the same potential and frequency as the measuring probe. Because current cannot flow between equal potentials, the measuring probe
Capacitance probes typically are coated with PFA (shown), Kynar, or polyethylene
does not sense material build-up between the probe and vessel wall.
Typical insertion lengths of standard capacitance probes range from 7 to 16 in. These probes typically are side-mounted (Figure 8-4A). Vertical probes can be extended by solid rods up to a length of 1.2 to 1.5 m (4 to 5 ft), or a steel cable with a weight can be
Figure 8-4: Click on figure to enlarge.
used to suspend the probe up to 15 m (50 ft) (Figure 8-4B). Most capacitance level sensors are provided with 3/4 to 1- 1/2 in NPT mounting connectors. The matching female coupling is usually welded to the vessel wall and the capacitance probe is screwed into the mating connector. Low profile capacitance sensors also are available (Figure 8-4C) and are flange-mounted.
In applications where the vessel is non-conductive and unable to form the return path for the RF signal, a second probe placed parallel to the active one or a conductive strip can be installed.
Electronics & Housings
The electronic circuitry of the probe performs the functions of: 1) rectifying and filtering the incoming power, 2) generating the radio frequency signal, 3) measuring the changes in current flow, and 4) driving and controlling interface devices such as relays, analog signal generators and display meters. The circuitry is usually of solid state design and provided with potentiometer adjustments for setting sensitivity and time delays.
Because the level sensor will ultimately drive an external device, it is advisable to evaluate for system compatibility the number of relays required, their capacities, or the analog signals required, time delays, and power supply requirements. More advanced microprocessor-based units are self-calibrating; sensitivity and time delay adjustments are under pushbutton control. These units are often supplied with self-test capability and built-in temperature compensation.
The more advanced designs are also two-wire, intrinsically safe, and supply your choice of standard 4-20 mA or digitally enhanced output using the HART (Highway
Table 7: Click on figure to enlarge.
Addressable Remote Transducer) protocol. Accuracy (including linearity, hysteresis, and repeatability, but excluding temperature and supply voltage effects) is typically 0.25% of range. Minimum span is 4 pF, and the upper range limit (URL) is 2,500 pF.
Level switches are usually provided with time delays for filtering out false readings caused by material shifts or splashing liquids. In addition, the feature of failsafe selectability provides a predetermined state for the relay output in the event of a power failure or malfunction.
Sensor housings are typically made from cast aluminum, steel, or synthetic materials such as glass-reinforced nylon. Most housings are suitable for outdoor installations in dusty or wet environments.
The Dielectric Constant
The dielectric constant of the process material is the most important aspect of the process data. The higher the difference between the dielectric constants (of the process material and the vapor space or between the two layers in the case of an interface measurement), the easier the measurement. If the difference is low (K2-K1 < 1.0 in Figure 8-2), a high sensitivity design (0.5 pF) must be used.
Each sensor has a capacitance
threshold, defined as the amount of capacitance change required to cause a change in the sensor output. The dielectric constant of a material can change due to variations in temperature, moisture, humidity, material bulk density, and particle size. If the change in dielectric constant results in a greater capacitance change than the calibrated capacitance threshold of the sensor, a false reading will result. This condition can usually be corrected by reducing the sensitivity (increasing the capacitance threshold) of the sensor.
As shown in connection with Figure 8-3, sensitivity can be increased by increasing the probe length (A) or by decreasing the size of the gap (D). Either or both changes will minimize the effect of dielectric constant fluctuations or increase sensitivity to low dielectrics. It is usually more practical to specify a longer probe than to decrease the distance (D) from the vessel wall. When the probe is installed from the side (Figure 8-4A), D is fixed, whereas if the probe is inserted from the top of the tank, D can be changed (if other considerations permit) by moving the probe closer to the wall of the vessel.
If the same vessel will hold different materials at different times, the capacitance sensor must be
Figure 8-5: Click on figure to enlarge.
equipped with local or remote recalibration capability.
Light density materials under 20 lb/ft3 and materials with particle sizes exceeding 1/2 in. in diameter can be a problem due to their very low dielectric constants (caused by the large amount of air space between particles). These applications might not be suited for capacitance-type level measurement.
Application Considerations
Materials that are conductive (water-based liquids with a conductivity of 100 micromhos/cm or more) can cause a short circuit between a bare stainless steel probe and the vessel wall. As the liquid level drops, the probe remains wetted, providing a conductive path between the probe and the vessel wall. The faster the level changes, the more likely this false indication is to occur. It is advisable to use PFA or Kynar insulator coating on the conductive probe surface when the process fluid is conductive.
Temperature affects both the sensor components inside the vessel (active probes and insulators) and the electronic components and housing outside. An active probe is typically made from stainless steel and, as such (unless it is coated), it is suitable for most applications. Probe insulators can be PFA, Kynar, or ceramic, and should be selected for the operating temperature of the application. The housing and the electronics are affected by both the internal and external vessel temperatures.
Ambient temperature limits usually are specified by the manufacturer, but heat conduction from a high-temperature process is more difficult to evaluate. Heat conduction can be reduced by using an extended mounting coupling or one made of a low thermal conductivity material. If such methods are insufficient, the electronics may be mounted up to 20 ft away and connected via coaxial cable. The cable's inherent capacitance, however, reduces the overall sensitivity of the system.
Housings must also be compatible with the requirements for hazardous, wash-down, wet, and/or dusty environments. Explosion-proof environments may require the housing to be certified. In addition, the active probe might need to be intrinsically safe.
If the process material is corrosive to stainless steel, the probe should be coated with Kynar or PFA for protection. Ryton is a good choice for abrasive materials, and, for food grade or sanitary applications, stainless steel and PFA are a good probe-insulator combination.
Installation Considerations
The capacitance probe should be mounted in such a way that its operation is unaffected by incoming or outgoing material flow (Figure 8-5A). Material impacts can cause false readings or damage to the probe and insulator. When measuring low-dielectric materials, it's important that the entire probe be covered, not just the tip (Figure 8-5C). When rod or cable extensions are used, allow for 8-12 in. of active probe coverage.
Install the probe so that it does not contact the vessel wall (Figure 8-5B) or any structural elements of the vessel. If a cable extension is used, allow for swinging of the cable as the material level in the vessel rises, so that the plumb bob on the end of the cable does not touch the vessel wall. The probe should not be mounted where material can form a bridge between the active probe and the vessel wall. In addition, the probe should not be mounted at an upward angle (Figure 8-5D), to avoid material build-up.
If more than one capacitance level sensor is mounted in the vessel, a minimum distance of 18 in. should be provided between the probes (Figure 8-5E). Closer than that and their electromagnetic fields might interfere. If a capacitance probe is installed through the side wall of a vessel and the weight of the process material acting on the probe is sometimes excessive, a protective baffle should be installed above the sensor (Figure 8-4A).