Measuring temperatures inside a furnace can present several challenges: high temperatures, temperature cycling, and hostile atmospheres exceeding the limits of many measurement devices while others have greatly reduced lifetimes and poor accuracy.
This article approaches mainly two specific challenges related to temperature measurement in furnaces: types of oxidizing and reducing atmospheres in furnaces used in microelectronics fabrication.
Furnaces Overview
The need to heat is common to many manufacturing processes. Rubbers and adhesives are cured, metals are annealed to modify their metallurgy and properties, coatings are dried, metals are melted, and ceramics are fired or vitrified. Many of these processes are carried out in ovens, heated either by electricity or gas. An oven that can heat to above 1000°C (1832°F) is termed a furnace.
A kiln is a particular type of furnace used in ceramics. At high temperatures many materials start to react with the surrounding atmosphere. If that atmosphere is very short of oxygen, it may pull oxygen from the material being heated. Such an atmosphere is termed “reducing”. Gas heating usually results in an oxygen-deficient atmosphere. If the atmosphere is oxygen-rich, the material being heated will capture a proportion, forming an oxide layer. Such an atmosphere is termed “oxidizing.” This is the process employed in diffusion furnaces used in microelectronics fabrication to produce SiO2.
Electrical heating is more likely to produce an oxidizing atmosphere. Control of the atmosphere can be achieved in several ways. Gas may be piped into the chamber, which might be done to create an inert atmosphere. Alternatively, a vacuum furnace could be used.
High Temperature Measurement Options
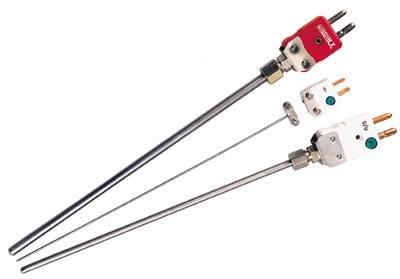
The upper limit for thermistor devices is around 100°C (212°F) and RTDs are limited to around 750°C (1382°F). That leaves thermocouples and infrared pyrometers or imagers as the most suitable devices for measuring temperatures above 1000°C (1832°F).
Thermocouples
Thermocouples utilize the Seebeck effect (the difference in EMF between dissimilar metals) to produce a signal proportional to temperature. Nickel-chromium and nickelalumel are the metal pairs most commonly used in what is called the “Type K” thermocouple.
The Type K is inexpensive and can be used across a temperature range from -200 to 1250°C (-328 to 2282°F). However, metallurgical changes at temperatures above 1000°C (1832°F) reduce accuracy, and cycling through this temperature induces hysteresis effects, further reducing accuracy. Type K thermocouples are also vulnerable to corrosion in an oxidizing atmosphere.
Thermocouples can be damaged or fail in-service, requiring replacement. If this entails shutting down and cooling a continuous furnace it can be a difficult and expensive undertaking. For this reason it’s common to include redundant thermocouples throughout the heating chamber.
IR Pyrometry
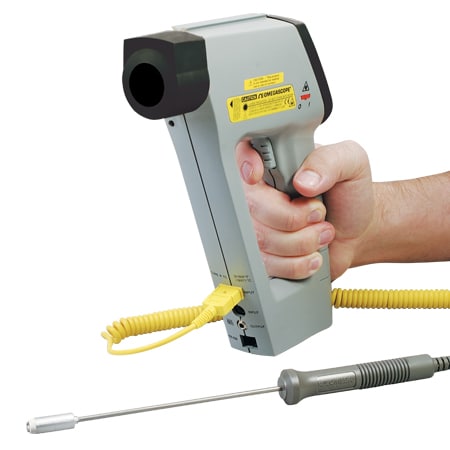
Infrared (IR) pyrometry presents a convenient contactless method of measuring high temperatures. This technology takes advantage of Planks Law, whereby the wavelength and intensity of the IR radiation emitted from a surface is proportional to its temperature. A pyrometer or thermal imager detects this radiation, converting the signal to a temperature.
IR pyrometry works well when the surface of the hot material is exposed, as with molten metal in a ladle. Using it to measure temperatures inside a furnace is more difficult, as it needs to be viewed through a window. This window must transmit IR radiation of the wavelength corresponding to both the sensitivity of the detector and the temperature being measured.
Regular glass is opaque to some IR wavelengths, particularly between six and seven microns. Chalcogenide glass is manufactured especially for IR transmission applications but is limited to temperatures below around 370°C (698°F). Sapphire is an alternative window material that transmits wavelengths up to four microns but is relatively soft and easily damaged. When a sapphire IR window is used as a viewing port it should be designed without any projections that would make it vulnerable to damage. Sapphire also has a temperature limit of around 450°C (842°F), making it unsuitable for furnace applications.
Emissivity is always an issue with pryometry: different materials at the same temperature radiate different intensities of IR radiation and the sensor must be calibrated for this. The window will have an influence on the radiation transmitted.
High Temperature Thermocouples
Two families of thermocouples are available, those using tungsten-rhenium junctions and those of platinum-rhodium. The tungsten-rhenium thermocouples, (Types G, C and D) operate at temperatures as high as 2320°C (4208°F) but will not survive an oxidizing atmosphere.
For oxidizing atmospheres platinum-rhodium thermocouples, sometimes referred to as “noble metal thermocouples,” should be selected. These are available as Type R, [maximum of 1460°C (2660°F)] S, [maximum of 1450°C (2642°F)] or B, [maximum of 1700°C (3092°F)]. They are more expensive than base metal thermocouples.
Sheaths for Thermocouples
Depending on the installation, it’s common to protect thermocouple wires by placing them inside a protective tube or sheath. Stainless steel is widely used as it is inexpensive and resists corrosion. However, it has a melting point of around 1400°C (2552°F), limiting service temperature to under 1100°C (2012°F) and reacts with oxidizing atmospheres.
For highest temperature capabilities, consider using either tantalum or molybdenum sheaths. These will go up to 2315°C (4199°F) and 2200°C (3992°F) respectively, although both are sensitive to oxidation, so should not be used in oxidizing atmospheres. The alternatives are ceramic sheaths, which will withstand up to 1960°C (3560°F), platinum-rhodium alloy sheaths, which will withstand 1650°C (3002°F), or Inconel® 600, which goes up to 1150°C (2102°F). All of these can handle oxidizing atmospheres.
Sheath Materials
Code | Material | Max Operating Temp | Working Environment | Approx Melting Point | Remarks |
XTA | Tantalum | 2300°C 4200°F | Vacuum | 3000°C 5425°F | Resists Many Acids and Weak Alkalies. Very Sensitive to Oxidation Above 300°C (570°F) |
XMO* | Molybdenum | 2200°C 4000°F | Inert Vacuum Reducing | 2610°C 4730°F | Sensitive to Oxidation Above 204°C (400°F) Non-Bendable |
XPA | Platinum-Rhodium Alloy | 1650°C 3000°F | Oxidizing Inert | 1870°C 3400°F | No Attack by SO2 at 1093°C (2000°F). Silica Is Detrimental. Halogens Attack at High Temp |
XIN | Inconel 600 | 1150°C 2100°F | Oxidizing Inert Vacuum | 1400°C 2550°F | Excellent Resistance to Oxidation at High Temp. Hydrogen Tends to Embrittle. Very Sensitive to Sulfur Corrosion |
*Refractory metals are extremely sensitive to any trace of oxygen above approximately 260°C (500°F).
Thermocouple Insulation
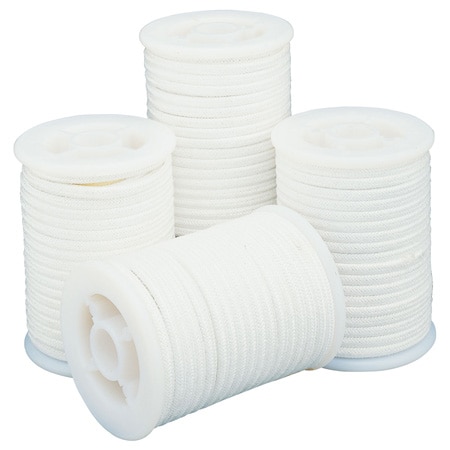
Insulation is incorporated into a thermocouple sheath to keep the wires from contacting the sides. This insulation must have a temperature rating appropriate to the environment. Common materials for furnace temperatures are alumina, magnesia, and hafnium oxide. Alumina has a maximum temperature rating of 1540°C (2804°F) while magnesia and hafnium oxide will go to 1650°C (3002°F) and
Takeaways
Thermocouples are a good option for measuring temperatures inside furnaces. While the widely-used “Type K” thermocouples will handle furnace temperatures, better performance is offered by Types G, C and D and R, S and B. At furnace temperatures, the type of atmosphere employed becomes an important consideration. In particular, an oxidizing atmosphere, as used in microelectronics fabrication, will cause a reaction with both Types G, C and D and the stainless steel sheaths often employed.
IR pyrometry is an alternative for measuring high temperatures, but requires a viewport or window to measure inside a furnace. For this reason it is generally preferred when there is an uninterrupted line-of-sight.